Advancing the Management of Genetic Diversity Ex Situ
Botanic gardens and seed banks have incredible capacity to conserve the diversity of plant life in their living collections. For instance, botanic gardens globally house millions of specimens from over 100,000 plant species, including many of cultural, horticultural, and economic importance (Mounce et al 2017). Such collections have the greatest value to researchers, breeders, and conservationists when they are well-documented, genetically diverse and representative, and managed in a coordinated fashion across institutions (Griffith et al 2019).
Why conserve genetic diversity? Genetic diversity is vital for supporting the resilience (able to return to steady state after shock) and adaptation (able to evolve to a new state as needed) of species in the face of changing climate and pests and diseases, and for successful ecological restoration (Hoban et al 2021, 2022). Genetically representative means conserving the genetic, trait, and ecological breadth across plant species’ native ranges (Guerrant et al 2014).
In this article I will explain a series of recent advances that have helped guide and improve how we conserve genetic diversity in botanic gardens. These include: assessing how much genetic diversity is represented in botanic gardens, assessing how much ecogeographic diversity is represented in botanic gardens, and determining how to more effectively sample seeds from wild populations for future botanic garden collections.
How much genetic diversity have we conserved? Recently, DNA studies have quantified how gardens are progressing towards safeguarding genetic diversity of species. How does this work? These studies involve (1) collecting leaf tissue samples from wild populations and as many botanic gardens as possible that are growing the target species, (2) analyzing the genetic diversity, and (3) calculating what percentage of the wild genetic diversity is actually in gardens today. The studies so far show that the percentage conserved varies a lot among species (from 28% to 95% in Hoban et al 2020). At least one clear message has resulted – we need more plants in gardens (as noted by Heywood 2009; Marshall & Brown 1975), usually more than a hundred and often several hundred plants per species to conserve the majority of rare plant species’ genetic diversity (Hoban 2019)!
Figure 1: We quantify the percentage of genetic diversity conserved in botanic gardens by sampling from wild populations and botanic gardens and performing DNA based analysis.
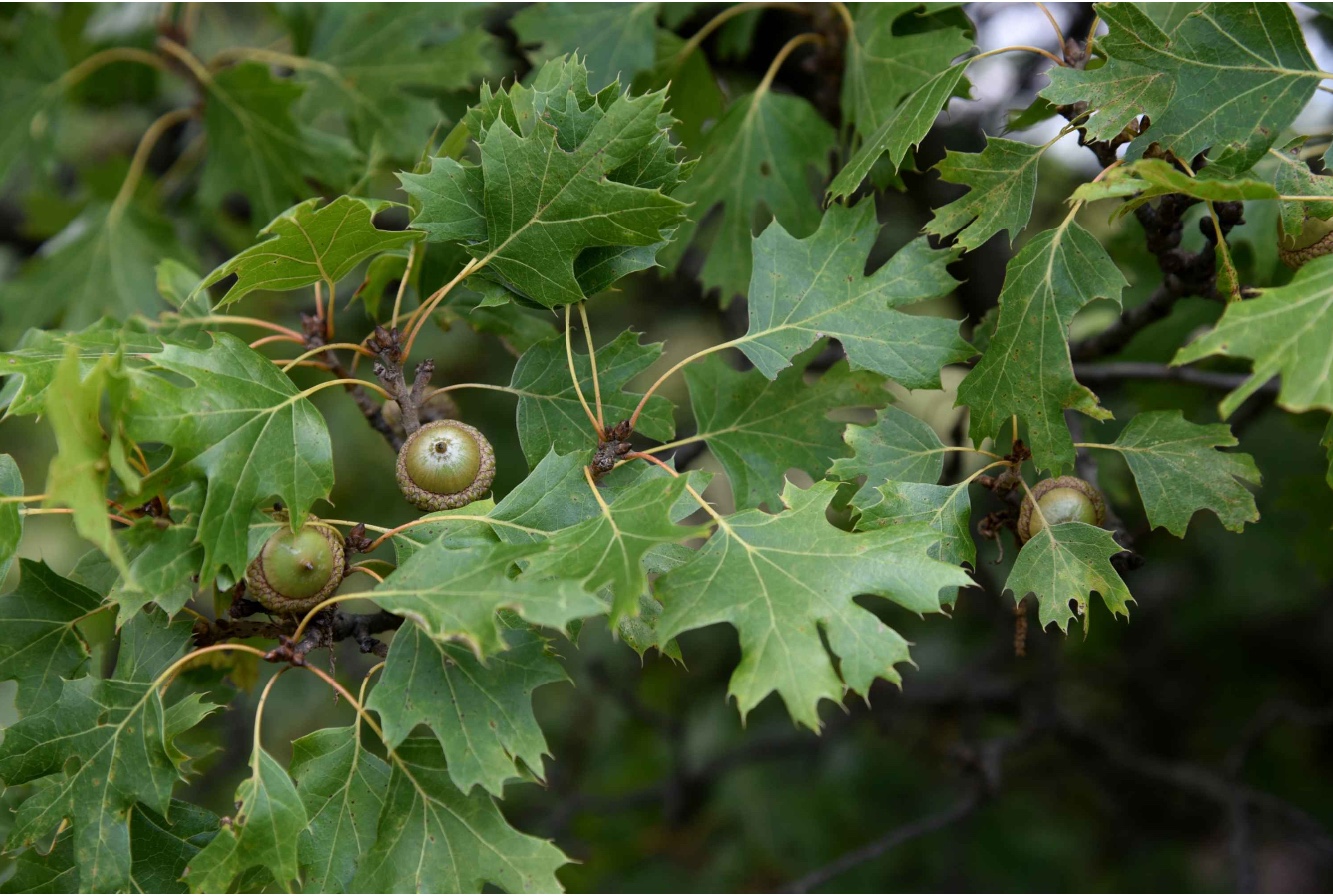
I will highlight several examples. Quercus acerifolia is a rare oak with only four wild populations whose genetic diversity is very well-conserved (95 to 98% of total genetic diversity) in 277 ex situ plants (a larger ex situ collection than most rare plants). Diversity is even “backed up,” with more than 88% of alleles in at least 3 copies, meaning the collection is resilient to a moderate degree of loss (an important issue, see Griffith et al 2017). Another well conserved species is Zamia lucayana, endemic to the Bahamas, with 91 to 95% of its diversity conserved in 244 plants. This data helps to evaluate if species are ‘sufficiently’ conserved – and unfortunately we find that most species (11 of 15 we’ve studied, unpublished data) are not sufficiently conserved. One example of a species whose genetic diversity urgently needs more conservation is Quercus hinkleyi, a Critically Endangered oak in Texas (only two very small populations) threatened by climate change. Only 22 individuals are safeguarded ex situ, with only 57% of diversity conserved. In addition, one of the two populations has never been sampled for seed to place in ex situ collections (Backs et al 2021).
Figure 2: Quercus acerifolia leaves and acorns.
How much ecogeographic diversity have we conserved? Meanwhile, several groups developed a methodology for analyzing geographic and ecological representation of our ex situ collections. How does this work? This method involves (1) creating a map of a species potential or likely wild distribution based on occurrence records (mostly from herbaria), (2) overlaying on that wild distribution the geographic points where species have been sampled and these samples are now in ex situ collections, and (3) calculating the area covered by those ex situ points or calculating the ecogeographic regions that are covered. This method has found that geographic diversity conserved ranges from 0 to 100% (on average, 40% for geographic and 59% for ecological, with high variation among species) for US oaks (Beckman et al 2019). We also applied this method to nine other US tree genera (for example, Beckman et al 2021), and it is being expanded to Magnolia and other groups. A series of papers applied a similar approach to many crop wild relatives, highlighting that such species are poorly conserved at present (Khoury et al 2020, 2019, among others). A very exciting development is to start to compare the extent of genetic conservation (from DNA studies) to ecogeographic conservation (from this GIS method). One such comparison exists, in Quercus havardii, a widespread threatened oak (Zumwalde et al 2022).
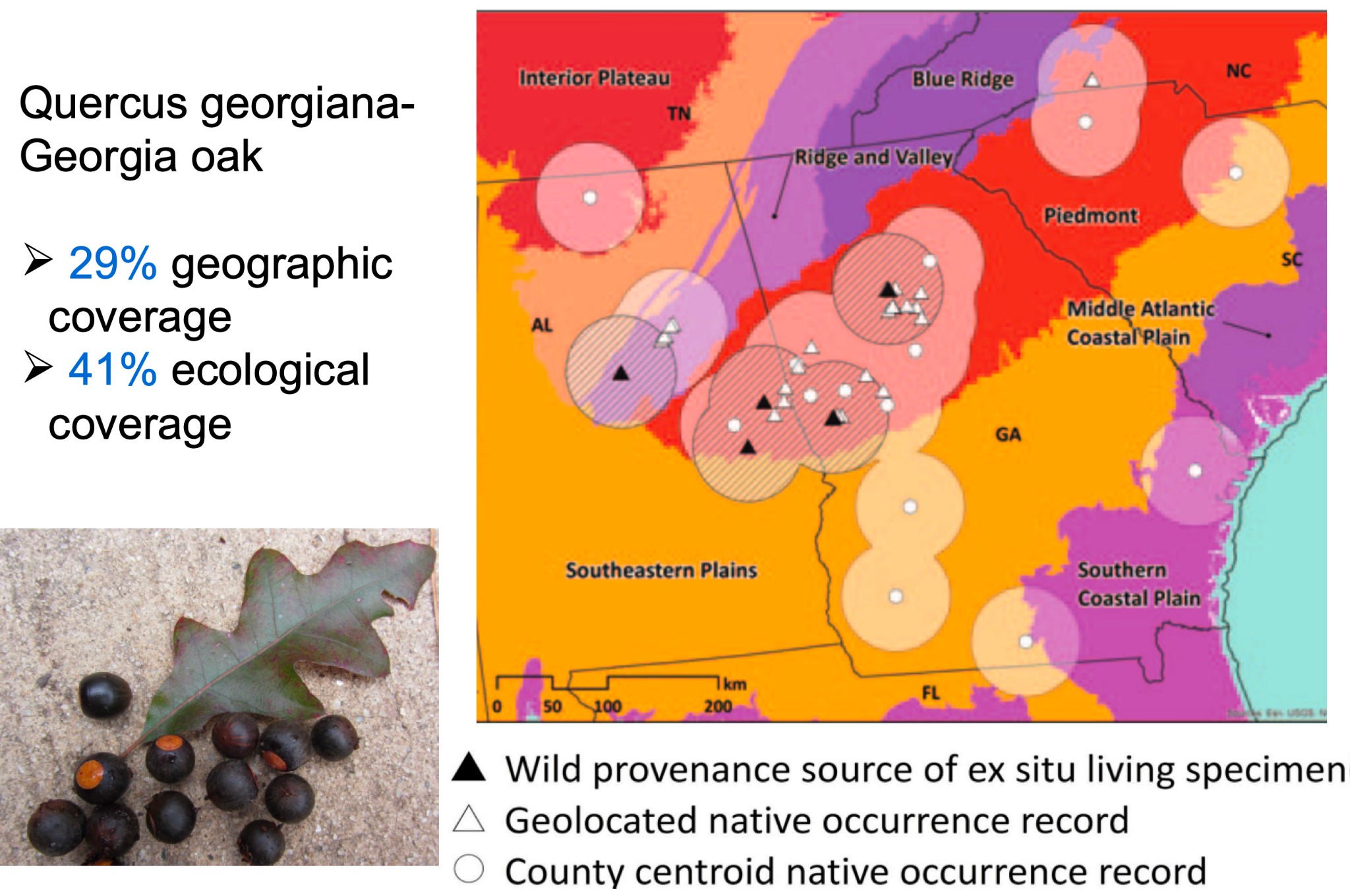
Figure 3: We can use maps like this one to calculate the percentage of species’ geographic area, or ecoregions a species occurs in, as a proxy for genetic diversity conservation.
How can we sample more efficiently? Studies have also focused on evaluating how we should sample per species, providing general guidance on minimum sample size, and on practical field advice. Early studies showed that (1) as species population ranges are more subdivided, samplers must allocate more sampling among populations (e.g. it is more important to reach the entire range), (2) species that have lower dispersal, higher selfing, and longer lifespans need more samples, (3) species with larger population sizes need more sampling, and (4) when sampling within populations, samplers should use grid or random sampling, rather than roadside or transect sampling (Hoban and Strand 2015, Hoban and Schlarbaum 2015). More recently, we showed that it is often, but not always, advantageous to sample proportional to the population size – more in larger populations and less in smaller populations (Rosenberger et al 2021) – but don’t neglect small populations entirely! We continue to investigate minimum sampling across the oak genus, and how pollen flow impacts genetic diversity in sampled seed – stay tuned for exciting results! Other researchers are also advancing the science of ex situ collections, by optimizing the size and composition of translocated populations to maintain genetic diversity over time, and to achieve other objectives, such as integrating the conservation of diversity and adaptive variation (Bragg et al 2021, 2022).
I close by noting that genetic diversity is on the discussion table for the Convention on Biological Diversity, whose final post-2020 commitments will be negotiated in December 2022 in Montreal. We must communicate and work with policy makers to ensure that CBD commits to conserving (in situ and ex situ), protecting (with policies and law), managing (such as through genetic rescue, restoration, and connectivity), and monitoring (using genetic and non genetic methods) genetic diversity of all types of species, to benefit humans and nature (Hoban et al 2020, Laikre et al 2020, O’Brien et al 2022). CPC and partners can help with such translation of science to policy.